Neutral Beam Splitters
A beam splitter is a device designed to divide a beam of light into two parts, usually a transmitted and a reflected portion. Neutral beam splitters aim to provide constant reflectance and transmittance over a specified wavelength range, making them ideal for applications requiring even light division. This section examines neutral beam splitters, focusing on metallic and dielectric designs.
1. Beam Splitters Using Metallic Layers
Metallic beam splitters, the simplest type, consist of a thin metal film on a glass substrate. Silver, known for its low absorption in the visible region, has traditionally been popular, although modern beam splitters often use chromium for greater durability.
Characteristics:
- Metallic films absorb light, reducing efficiency.
- Transmittance remains constant regardless of direction, while reflectance varies depending on the medium (air or glass).
- Absorption is higher when light reflects from the glass side due to reduced reflectance.
Enhancements:
- Adding a dielectric layer can reduce absorption, as demonstrated with chromium films. A quarter-wave zinc sulfide layer significantly lowers reflectance at the glass–film interface, enhancing transmission efficiency.
- Antireflection coatings, both dielectric and metallic, can further improve transparency and reduce absorption. For example, a thin silver layer on a heated substrate increases the transparency of an aluminum film.
Limitations:
Metallic beam splitters often exhibit polarization-dependent reflectance. The s-polarized reflectance (TE) is typically higher than the p-polarized reflectance (TM), especially at oblique angles of incidence.
Cube Beam Splitters:
To address issues like rear surface reflections and aberrations, beam-splitting cubes are used. However, these configurations experience higher absorption because both surfaces are in contact with a higher-index medium.
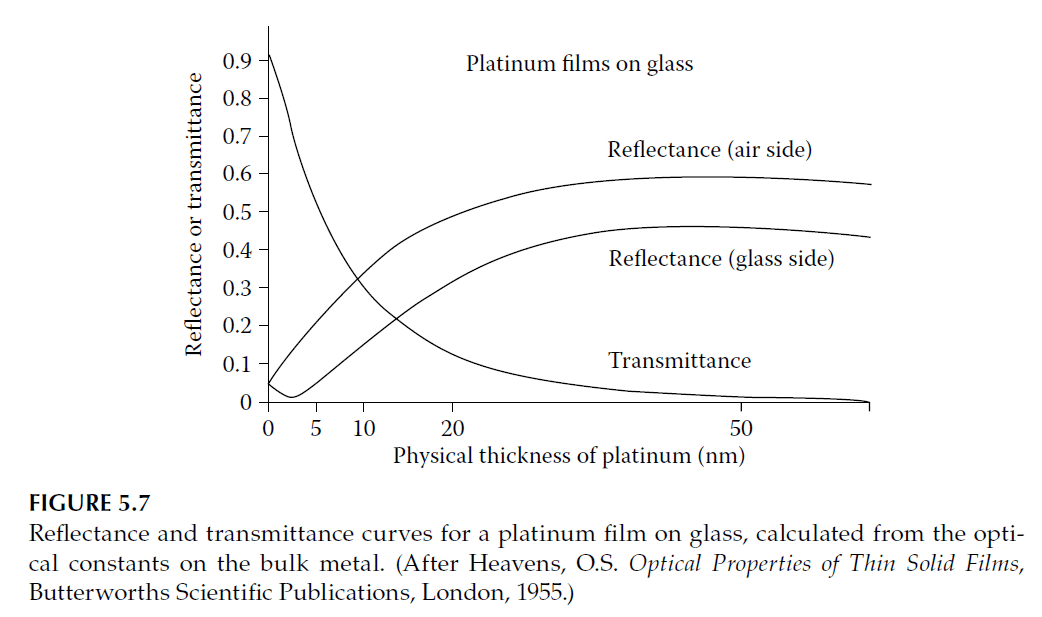
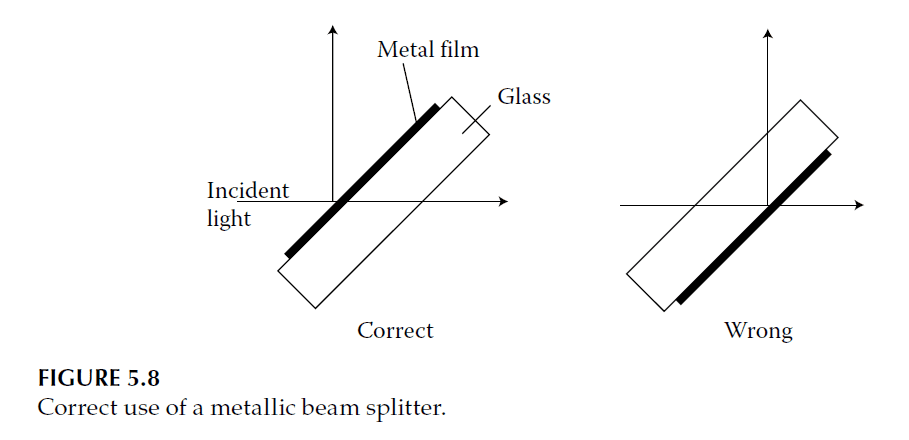
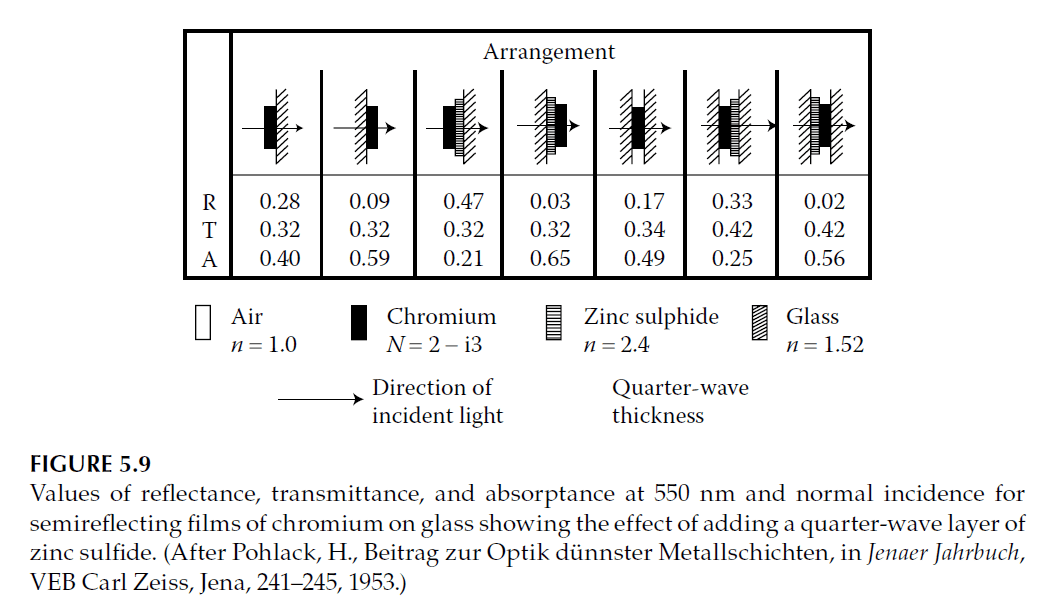
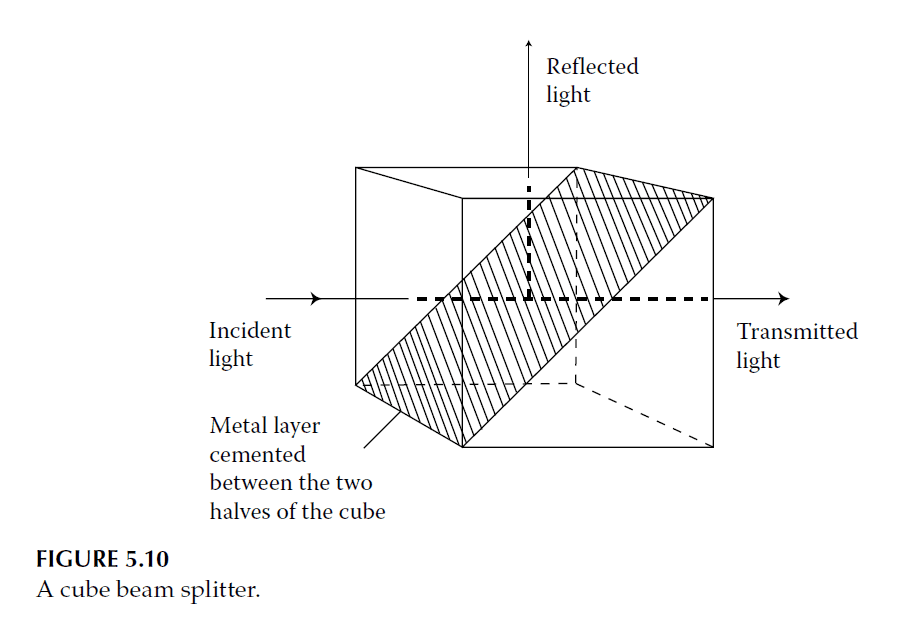
2. Beam Splitters Using Dielectric Layers
Dielectric beam splitters use transparent high-index materials instead of metals, offering better performance for some applications.
Characteristics:
- At normal incidence, the reflectance of a high-index quarter-wave layer is calculated as: \[
R = \frac{(\eta_0 – \eta_{\text{sub}})^2}{(\eta_0 + \eta_{\text{sub}})^2}
\] - At 45° incidence, peak reflectance shifts to shorter wavelengths, and reflectance values differ for s- and p-polarized light.
Materials:
Zinc sulfide (\(n = 2.35\)) is commonly used, providing good reflectance and transmittance characteristics. Titanium dioxide (\(n \approx 2.8\)) or cerium dioxide (\(n \approx 2.25\)) are robust alternatives for demanding applications.
Advanced Designs:
- Adding a low-index half-wave layer broadens the performance range, achieving flatter reflectance curves.
- Multilayer designs, such as Air | LHLH | Glass, achieve ~50% reflectance, which can be improved further by adding half-wave layers.
Cube Beam Splitters:
Dielectric coatings in cubes suffer from reduced performance for p-polarized light due to the higher effective angle of incidence. While metallic layers are often necessary for cubes, dielectric layers can be advantageous in polarizer designs.
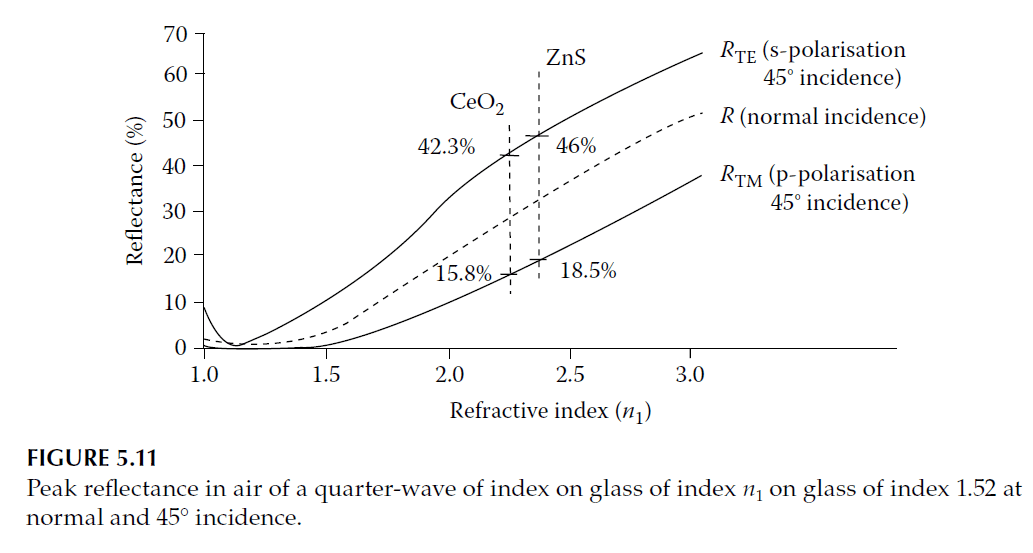
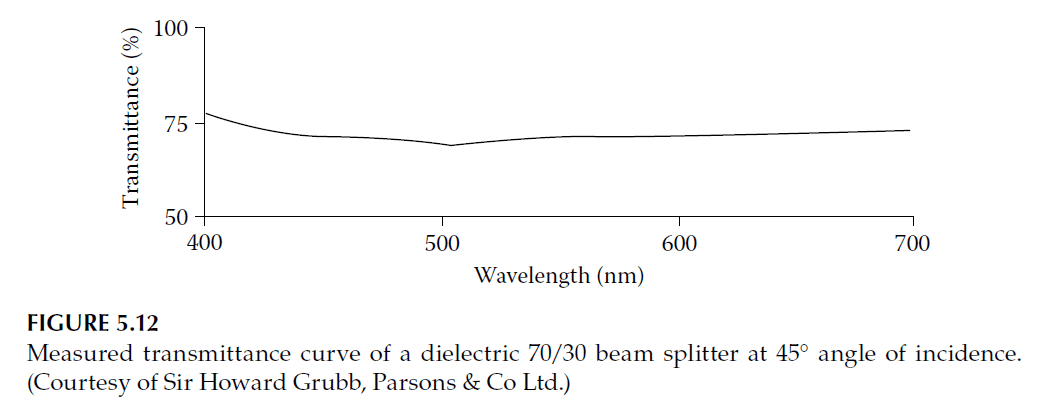
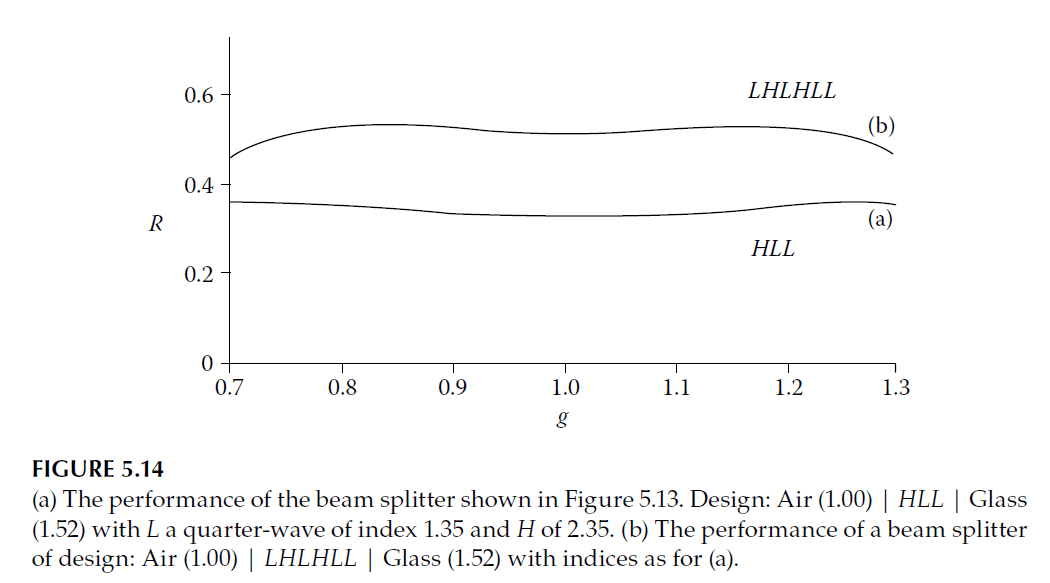
Challenges in Nonpolarizing Designs
Creating nonpolarizing dielectric beam splitters for oblique incidence remains challenging due to polarization differences and limited design flexibility with two materials. Adding a third material introduces slight improvements, though the problem remains complex. Advanced designs, often achieved through computational synthesis, offer solutions for narrow spectral regions.
References
- Figures and Data: Reflectance and transmittance curves for various beam splitters (Figures 5.7–5.14).
This tutorial demonstrates that while metallic beam splitters are straightforward and durable, dielectric designs offer better performance and efficiency for specific optical systems. Advanced multilayer and computational approaches continue to expand the capabilities of beam splitter technology.